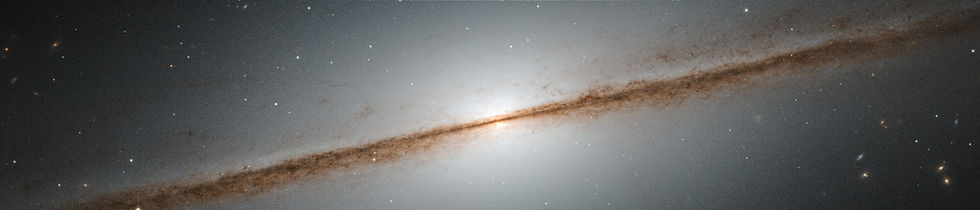
Galactic fountain and gas accretion
​
Star-forming galaxies grow through the continuous acquisition of gas from the surrounding environment (gas accretion). This accretion is fundamental for the formation of new stars throughout the Universal time. Despite multiple indirect evidence, the way this accretion takes place remains not fully understood and it is the subject of current investigation both theoretically and observationally.
Fountain accretion (also called fountain-driven or supernova-driven accretion) is a theoretical framework in which gas accretion onto galaxies takes place due to the cooling of the surrounding ambient gas. This cooling is triggered by the interactions between the ambient gas and disc gas that is ejected into the surrounding medium by stellar winds and supernova explosions (stellar feedback). These phenomena produce a cycle of gas from the disc to the ambient and back that is called galactic fountain.
​
Key observational starting points of the fountain accretion are:
-
The discs of spiral galaxies are surrounded by thick layers (several kiloparsec) of "cold" (T<10000 K) extraplanar gas, see figure below. The gas in these layers is too cold to be in hydrostatic equilibrium, thus must be continuously circulating from disc to halo and back (galactic fountain).
-
The regions surrounding the galaxy also contain gas at much higher temperature, the galactic corona, which is thought to extent to very large radii out to the virial radius (200-300 kiloparsec for typical disc galaxies today).
Inevitably, the galactic fountain gas must interact (mix) with the coronal gas and this produces exchanges of mass and momentum between the two components with radical consequences for the evolution of the galaxy.

Comparison between cold and hot gas in the halo region close to the disc of the edge-on galaxy NGC 891, the two images are on the same scale. a) Total H I map (blue) from Oosterloo et al. (2007) overlaid on an optical image (orange). b) Chandra X-ray observations of NGC 891 overlaid on an optical image (from Hodges-Kluck & Bregman 2013). Note that this is only the very inner part of the galactic corona, which likely extends, albeit with much lower densities (and therefore undetectable with these observations), out to the virial radius.
Analytic models and hydrodynamic simulations show that the interaction between "cold" fountain material and the hot coronal gas produces the rapid cooling of this latter, a process that has been called condensation. This can be see in the figure below.
As a consequence of this condensation part of the hot corona rains down onto the galactic disc providing new fresh gas that the galaxy can use to form new stars. It is conceivable that a galaxy like the Milky Way has acquired most of the gas to form stars (at least in the so-called thin disc) via this process. In particular, the Sun itself, being a relatively young star (about 5 billion year old, compared to the age of the Universe of 13.8 billion years), may have formed out of a mixture of recycled gas in the disc and gas accreted from the ambient medium via fountain accretion.
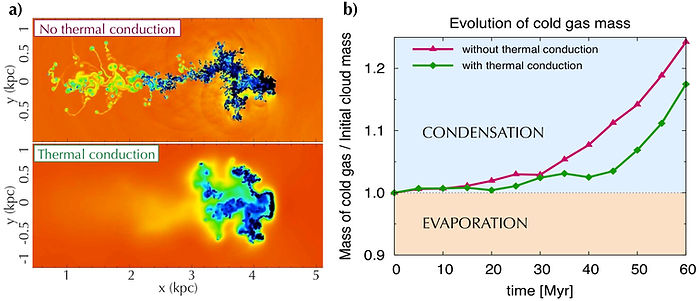
a) Temperature snapshots extracted after 60 million years from a hydrodynamical simulation of a cold (10000 K) fountain cloud moving (along the x-axis from left to right) through a hot (T=2 million K) and metal-poor (1/10 of the chemical elements of the Sun) medium. The top panel shows a simulation with radiative cooling akin to the one in Fig. 5, while in the bottom panel thermal conduction is also included. b) Behavior of the mass of cold (T < 20000 K) gas in the simulation box. The mass increases with time because of the condensation of the corona.
The fountain accretion model makes clear predictions that have withstood all observational tests so far. The main prediction is related to the kinematics of the extraplanar gas. The exchange of material between cold and hot gas produces several effects. The most important are:
-
transfer of mass mainly from the hot phase to the cold phase (condensation) for galaxies like the Milky Way today (but likely the opposite for more massive galaxies);
-
transfer of momentum that produces a reduction in rotation velocity of the cold gas (vertical gradient, ubiquitously observed in the extraplanar gas layers);
-
formation of intermediate temperature gas generated by the mixing and by the condensation that can be used to further test the model, see figure below.
A final prediction that distinguish this model from most of the other current models is that gas accretion occurs on top of the star forming disc while all most recent cosmological simulations predict accretion at the edge of galaxy discs. This is in principle testable.

a) A sketch of a fountain cloud, ejected from the disc by supernova feedback and interacting with the hot gas in the corona. In the turbulent wake, coronal gas mixes with high-metallicity disc material triggering the condensation of a fraction of the corona, which is then accreted onto the disc. An observer looking toward a background source intercepting the wake detects absorption lines from the ionised intermediate-temperature material. An observer looking toward the cold front detects HI emission. b) Predicted locations (darker shades show higher probability regions) in longitude and velocity of the intermediate-temperature material based on the fountain accretion model. The points show the HST/COS absorption features detected in the temperature range 4.3 < log(T/K) < 5.3. The vast majority (94%) of this ionised gas is consistent with being generated in wakes of fountain clouds.